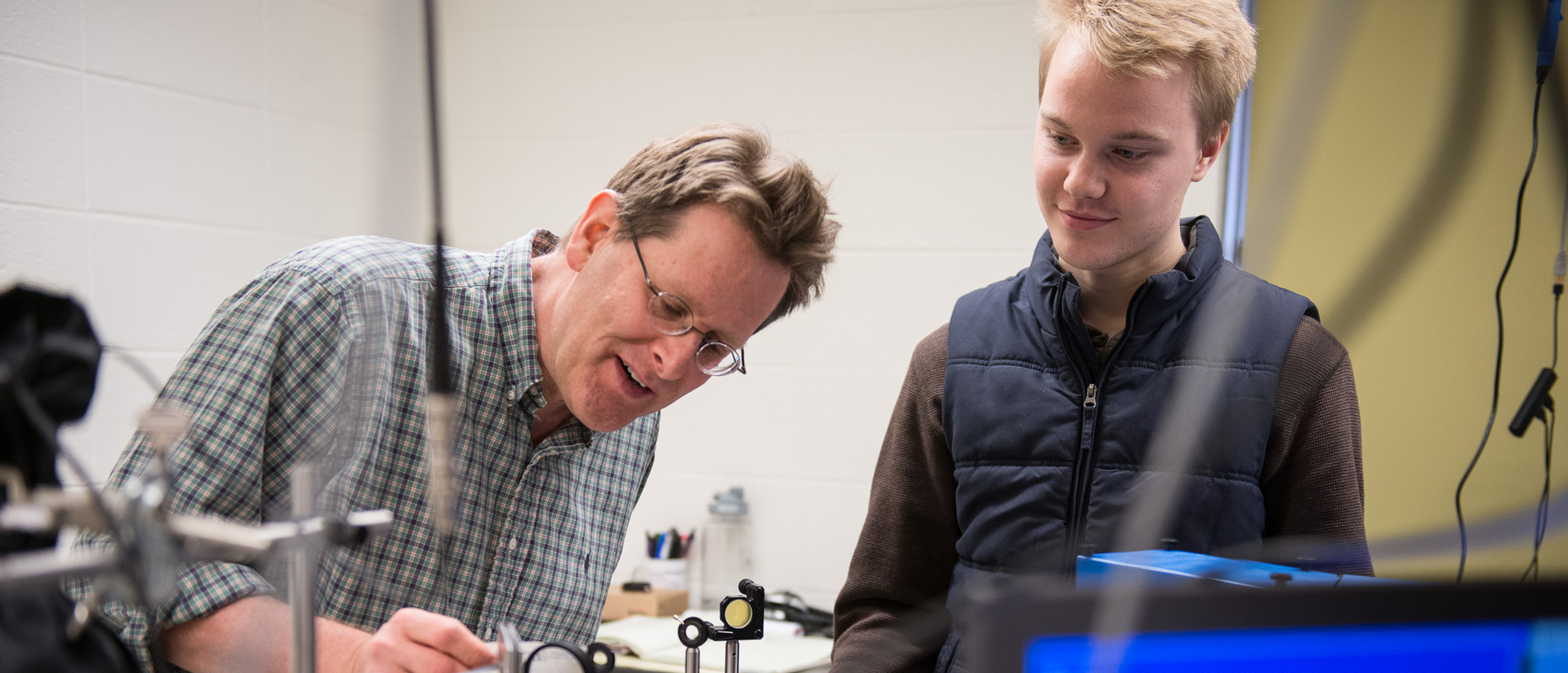
Research in Chemistry and Biochemistry
Center of Excellence
As the designated UW-System Center of Excellence for Faculty and Undergraduate Student Research, UW-Eau Claire has a lot to live up to, and chemistry is one of the pillars of that excellence. UW-Eau Claire’s chemistry and biochemistry department have an established record of quality undergraduate instruction that begins with well-developed courses taught entirely by Ph.D. chemists and builds on this solid foundation by involving students in collaborative research projects.
In chemistry, the myriad of research opportunities stems from a core group of young and enthusiastic faculty with diverse interests, together with senior faculty who have a record of sustained funding from external agencies. Over the last ten years our chemistry faculty has attracted over 4 million dollars in external funding to support student-faculty collaborative research on campus. A key facet of the Blugold undergraduate research experience in chemistry is the opportunity for hands-on use of an impressive array of modern instrumentation — this state-of-the-art instrumentation is used almost exclusively by undergraduates for coursework and research projects, and this experience leaves our students exceptionally well prepared for graduate studies and/or careers in industry.
In the past decade, these research experiences have resulted in over 130 of our students appearing as co-authors on articles published in peer-review journals, and a similar number presenting their research results at national scientific meetings. These high impact outcomes help to explain why UW-Eau Claire ranks in the 97 percentile among its peer institutions as the baccalaureate origin of students who go on to earn a Ph.D. in chemistry.
One of the highlights of my undergraduate career was performing cutting-edge research with Dr. Jim Phillips of the chemistry department. Because of our research, I presented at 2 national research conferences on both coasts of the USA, published scientific articles in scholarly journals, and graduated with departmental honors. These experiences set me apart from other students when applying for graduate schools and were key for my acceptance and fellowship offers in top programs.
Funding for student research
Perhaps most importantly, the University's administration is committed to promoting research as an important element of undergraduate education, and has maintained its support through a period of major cuts in state funding. The UWEC Office of Research and Sponsored Programs (ORSP), supports faculty-student collaborative research projects across all disciplines via internal grant programs that provide funds for student stipends during the summer and academic year, modest summer stipends for faculty, as well as travel. In the past decade, over 480 thousand dollars of funds from these grant programs have been channeled as stipends to students carrying collaborative research in chemistry.
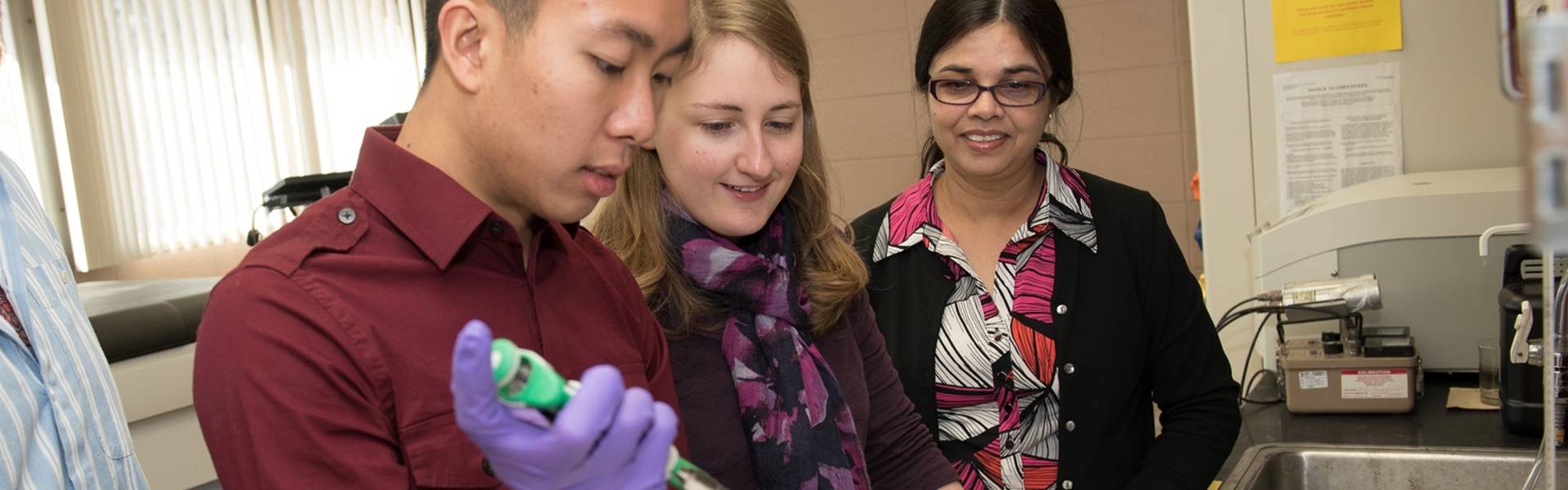
Ongoing research in the chemistry and biochemistry department
What our chemistry students and alumni tell us time and again is that the opportunities to conduct and present sophisticated collaborative research with faculty is the factor that makes this undergraduate program outstanding. See the categories below for a description of each area of ongoing research in the department, and contact the professors named if you are interested in joining a research team.
Physical Chemistry Research
Research activities in Steve Drucker's group are carried out collaboratively with 2-5 undergraduate students each year. Supported by National Science Foundation, we conduct our work within the discipline of physical chemistry. This area of chemical inquiry uses established models from the field of physics to analyze and predict the behavior of chemical entities (e.g. atoms and molecules) undergoing reactions. The work of Drucker's research team is focused on improving our understanding of photochemical reactions. During photochemical events, energy from the sun or other light sources is used to weaken targeted chemical bonds in a molecule and thereby promote reactions that could not occur at a reasonable rate under normal lab conditions.
The key event in photochemical reactions is a process known as electronic excitation. In a laboratory environment, we accomplish electronic excitation by exposing a sample of molecules to the light from a high-energy laser. Through absorption of laser energy, the negatively charged electrons in a molecule are pulled away from the positively charged atomic nuclei (i.e., protons) to which they are naturally attracted. The attraction between the electrons and the nuclei—which forms the basis for chemical bonding—is disrupted, or weakened, in the process of electronic excitation. This makes molecules more susceptible to the bond-breaking that underlies nearly every chemical reaction.
To predict the course of photochemical reactions, it is therefore desirable to learn how extensively the chemical bonds within an electronic excited molecule have been compromised. We are achieving this goal by using the experimental technique of laser spectroscopy. In the experiment we use a gaseous sample of the chemical compound under investigation. The sample, contained in a transparent glass tube, is exposed to a beam of laser light directed through the tube. We measure the amount of the laser light absorbed by the sample. We can also vary the wavelength (i.e., color) of the light to determine the dependence of light absorption on wavelength. We analyze this information by using well-established mathematical constructs that have been developed within the physics community. The analysis tells us, with high numerical precision, the extent to which specific bonds in the molecule are weakened in the photoexcitation process.
This information is immediately useful to investigators who wish to predict photochemistry via computational modeling. This information has great value for helping to design photochemical reactions with the greatest product yields and minimal waste.
See student-coauthored articles
Molecular Crowding and Enzyme Functions
The intra-cellular space is crowded with small and large biomolecules. How does an enzyme function in a crowded intracellular environment? Professor Hati's research group explores these questions through several ongoing projects funded by the National Institute of Health.
Proteins are over-expressed and purified using molecular biology and biochemistry techniques. Site-directed mutations are carried out to monitor the effects of a specific residue or a segment on a protein's structure and function. Crowding effects on the structure, dynamics, and function of a protein are investigated in the presence of synthetic crowders and using biochemical assays and spectroscopic techniques such as intrinsic tryptophan fluorescence spectroscopy, saturation transfer difference-nuclear magnetic resonance (STD-NMR) spectroscopy, and atomic force microscopy. In addition, Blugold Supercomputers are used for simulating the protein motions in crowded environments via atomistic molecular dynamic simulations.
See the student-coauthored publications
Molecular research
Our research is concerned with the structural and energetic properties in “molecular complexes”; associations (strong weak or intermediate) between two otherwise stable molecules. Over the long term, we have sought to identify and characterize systems that undergo major structural changes in response to condensed-phase environments (e.g., gas-phase to solid or solution), and the primary effect is a dramatic shortening of the donor-acceptor bond. This is depicted at the right for two nitrile - BF3 complexes, in which the B-N distances contract from 2.01 or 2.47 Å to about 1.65 Å while transitioning from the gas phase to the solid state. Our work has been primarily concerned with the effects of bulk condensed-phase media (e.g., solvents, and so-called “noble gas matrices” – cryogenic samples of solid neon, argon, or neon), and we have obtained insight into structural changes via shifts in vibrational frequencies measured by infrared spectroscopy. In addition, we gain key insight from quantum-chemical computations (computer simulations of the bonding). From these we obtain structures and frequencies for the gas-phase complexes, and most importantly, energy profiles along the donor acceptor bond (for both the gas-phase complex and in hypothetical solvents). These data have enabled us to propose a generalized mechanism for medium-induced structural change.
Going forward, we will pursue new directions by following one specific avenue motivated by a practical consideration, and also engage in an overall broadening our scope in an effort to identify new types of systems peculiar with structural and energetic properties like those described above. Just recently, we have been investigating nitrile (-CN) complexes of Group IV (M=Si, Ge, Ti) acceptors (e.g., CH3CN–SiF4) and we have demonstrated that we can modulate the strength of the donor-acceptor interaction and enhance the extent of condensed-phase structural change by altering the atom(s) on the end of the CH3CN subunit (e.g., FCH2CN–GeF4). Now, we will turn to derivatives the of the MX4 acceptors (M=Ge, Si; X=F, Cl) in which we will replace one of the X atoms with an organic group (R=CH3-, or C6H5-). If we can similarly optimize the condensed-phase response in these systems, we may have identified a route to a new class of molecular machines. Because both the nitrile (-CN) and MX4 (or MX3R) subunits have bonds that point directly opposite the donor-acceptor linkage, this could enable one to embed this structural motif into a larger structure of some type (e.g., a liquid crystal, or molecular wire). This process is depicted at the right. The general idea is that these structures could be exploited to exert a force along the length of that structure, which would open up this chemistry to a variety of electro-mechanical applications.
Computational Chemistry and Biochemistry
The research is Bhattacharyay group is inspired by the development of theoretical physical chemistry and computational science. Combining the growth of data science the new field of "Computational Chemistry" is producing significant discoveries in several fields of chemistry research. Supported by National Science Foundation grants, recent computational studies that have been published in peer-reviewed Chemical Society Journals include redox chemistry in COVID-19, spectroscopy of molecules, enzyme catalysis and mutations, enzyme functions in crowded environment, protein motions and functions.
See our students-coauthored peer-review articles
COVID-19
DOI:10.1021/acsbiomedchemau.1c00040
DOI:10.1007/s10930-022-10065-6
DOI:10.1007/s10930-020-09935-8
Spectroscopy of Molecules
Enzyme Catalysis and Protein Dynamics
Organic synthesis
Development of new chiral organocatalysts
Many biologically active molecules exist as molecules that exist in right-handed and left-handed forms (we call them chiral). The two forms are known as enantiomers, and occasionally the "inactive" enantiomer is not actually inactive, but cats elsewhere instead. Two such molecules are Ethambutol and warfarin. We are now designing molecules for use as organocatalysts based the camphor molecule; the aromatic ring of the organocatalyst completely blocks access of external reagents to one face of the double bond of the iminium ion derivative, so that only one stereoisomer of the product is formed. The ability to do this is becoming critical for the modern pharmaceutical industry.
Air Quality for Unmanned Aerial Vehicle
Our understanding of distribution of atmospheric pollutants is constrained by the kinds of platforms available for monitoring small quantities of trace gases. Studies on the air quality around Lake Michigan have shown steep gradients across the mesoscale meteorological process of the lake breeze front which passes over land on a daily basis. Our understanding of high ozone near Lake Michigan can be better understood by determining vertical mixing of ozone which requires sending sensors aloft. The advancements of both small Unmanned Aerial Vehicles (UAV) and smaller ozone monitors has paved the way for designing and implementing a sensor package on a remotely piloted vehicle.
Students in my research group have been working on integrating a sensor package that can measure temperature, humidity, wind vectors and ozone from a UAV.This project requires students to calibrate sensors, program and assemble microcomputers that serve as small data-logging systems, develop a working knowledge of UAV control systems and remote data streaming so as to design a platform that will be robust. We work on this project with Dr. Joe Hupy from the Geography department for prototyping and testing.
Biochemistry research
Our research group studies the binding of mucin peptide molecules to antibody molecule. Mucin peptides are interesting and important because they have been proposed to be used as antigens against some cancers in the field of cancer vaccines. Mucin peptides are short peptides that have the same or similar amino acid sequences compare to active segments on the mucin protein found in tumor cells. During the synthesis, we first make the peptide chain by reacting one amino acid at a time to lengthen the chain by a technique called Solid Phase Peptide Synthesis. The peptide synthesis reactions occur in solution, but the growing peptide chain is anchored onto a solid support resin. This way we can clean the growing peptide chain by washing off all the non-reacting chemicals and contaminants.
Once we obtain the peptide, we study its structure and make assignments of all the hydrogen atoms on the peptide by two-dimensional NMR (2D NMR) spectroscopy. After we learn almost everything about each peptide, we then study to see if the peptide can bind antibody molecule that was expressed against the whole tumor mucin protein.
Synthesis and characterization of complexes
This project focuses on the development, synthesis and characterization of new bioinorganic model complexes that mimic the active site of quercetin dioxygenase. The goal of this research project is to understand more about the important role dioxygenase enzymes play in biological systems.
Students under my supervision will carry out all experimental work, which involves synthesizing, characterizing and studying the reactivity of the proposed compounds. In the process, all students will learn spectroscopic techniques as well as how to interpret spectra. The advantage of a synthetic project like this is that it compels undergraduate students to develop strong laboratory skills for those planning on going on to medical school, graduate school, or for employment as scientists.
Synthesis and characterization of "safe" fluorescent stains for DNA
For decades, the stain used to visualize DNA bands after gel electrophoresis has been ethidium bromide. However, the use of this stain is not without serious drawbacks: 1) the compound itself is not mutagenic, but there is good evidence that its metabolites are; and 2) in many states, ethidium bromide solution is regulated as hazardous waste, which highlights another problem with this stain. We are designing fluorescent compounds that can be used as safe replacements for ethidium bromide. These stains will be based on the 4-amino-1,8-naphthalimide fluorophore, which is known to be non-mutagenic, and that we have found to be stable to storage for over a decade (two of our patented dyes have passed this mark). We are now designing a dye that should bind to the minor groove of the DNA, like the antimicrobial agent, netropsin, or to the major groove, like the nucleic acid stain, methyl green.
Medical chemistry
Towards safer oral anticoagulants (Emiritus Professor David Lewis)
Warfarin is the longest-prescribed oral anticoagulant in use in the U.S. but it shows sufficient inter-patient variability and sensitivity to its activity that regular blood testing is required to ensure patient safety. In 2014, we received a U.S. patent for a compound that enhances the activity of warfarin when co-administered with the drug. We are now undertaking studies to allow us to elucidate what is going on, with a view to making warfarin a much safer oral anticoagulant.
History of chemistry
History of organic chemistry in Russia
For several years, I have had a strong interest in the history of organic chemistry in Russia. At this time, we are translating Markovnikov'sM. Khim. and Dr. Khim. dissertations from Russian. Neither of these works has been translated into my western language before. Two students accompanied me to Kazan, Russia, in June 2015 to obtain these works in electronic format, and we will be providing this digitized work to the Butlerov Museum of the Kazan School of Chemistry. Both students had completed a year of Russian before we left.
"Smart" biaryls research
In this project we are synthesizing and studying bridged biphenyls and terphenyls to understand if we can control dihedral angle reversibly, thus achieving a molecular switch. The dihedral angle is the angle between the two phenyl rings with the single bond as an axis. Fluorescence, color, and conductance are dependent on the dihedral angle of biphenyl compounds. These “Smart” Biaryls could be useful broadly as sensors, molecular electronic components, molecular machines and in other nanoscience applications.
Fluorescent compounds research
Anthocyanidins are common plant pigments based on the flavylium ion. They are what give many fruits and flowers their colors. Natural anthocyanidins have recently been explored as components of dye-sensitized solar cells. These types of solar cells would be much cheaper, flexible, and possibly more efficient than current silicon-based solar cells. We are exploring new synthetic dibenzopyryliums with the same goal in mind. These very colorful compounds have pH-sensitivity as well and should be very useful as fluorescent and colorimetric pH sensors.
Probes for Cell Organelle Visualization
We have developed a series of chemically stable and non-toxic fluorescent probes based on the naphthalimide chromophore. We have used the confocal microscope to confirm localization of our probes to cellular targets by comparison to commercial probes and by transformation of cell lines with RFP labeled proteins targeted to those regions. We plan to use our probes to understand antibiotic toxicity and effectiveness.
Designing Self-healing Liquid Crystals
Liquid crystalline displays are everywhere- phones, TVs- even the monitor you're reading this on. One common problem is they're very fragile: drop it wrong and you're done and you're out several hundred dollars- AGAIN! Our research involves using reversible bonds to make LC displays able to heal themselves. That way when a break or fracture occurs you can put it in a special oven or light chamber and the fracture will repair itself.
Atmospheric particulate matter
My research group has been studying the characteristics of atmospheric particulate matter sampled in a frac sand mine. The size and composition of atmospheric particles are important quantities to measure because they influence the adverse health effects from inhaling these particles. Of concern is the amount of respirable crystalline silica that can be generated in an active frac sand mine, so my students have worked on two parallel analyses using the X-ray Diffractometer (XRD) and the Scanning Electron Microscope (SEM) to quantify the amount of crystalline silica and to characterize what other geologic parent material ends up in the sampled particles.Students take the lead on preparing samplers for the field, collecting field data, testing calibrations and analyzing field samples.
Analytical Chemistry Research
Students who have an interest in analytical chemistry applications that are readily studied using techniques from the Chemistry Department’s instrumentation can do so in this group. Current research projects involve heavy metal contamination of the water systems using the Microwave-Plasma Atomic Emission Spectrometer (MP-AES). A past study looked at the active ingredients in green tea supplements using High Performance Liquid Chromatography (HPLC).